
A modern day, closed-loop Rockwell tester is pictured above. Source: Wilson Hardness

Pictured above: 16 samples under automatic test utilizing current automation technology. Source: Wilson Hardness
How important and useful is material and hardness testing? Consider the information provided and its significance in structural, aerospace, automotive, quality control, failure analysis and many other forms of manufacturing and industry. Determining these material properties provides valuable insight to the durability, strength, flexibility and capabilities of a variety of component types from raw materials to prepared specimens and finished goods.
Over the years, various methods for determining the hardness of materials have been developed and employed at varying levels of success. From early forms of scratch testing to sophisticated automated imaging, hardness testing has evolved into an efficient, accurate and valued material test method.
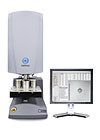
Pictured above: A modern-day, fully-automated microhardness tester. Source: Wilson Hardness
Scratch Testing
While testing techniques and hardware has significantly improved, particularly in recent years and in step with rapidly advancing electronics, computer, hardware and programming capabilities, earlier, basic forms of hardness testing, such as the simple scratch test, sufficed for the need of the relevant era.Some of the earliest forms of bar scratch testing date back to about 1722. These tests were based on a bar that increased in hardness from end to end. The level at which the material being tested could form a scratch on the bar was a determining factor in the specimens hardness.
Later, in 1822, hardness testing forms were introduced that included scratching material surfaces with a diamond and measuring the width of the resultant line, a test eventually known as the Mohs scale. In some processes this method is still utilized today.
The Mohs scale consists of 10 minerals, ordered from hardest at 10 (diamond) to softest at 1 (talc). Each mineral can scratch those that fall below it in the scale hierarchy. The Mohs scale is not linear; the difference in hardness between 9 and 10 is significantly more than that between 1 and 2. To put the Mohs scale into perspective, a tangible example is that of hardened tool steel which falls at approximately 7 or 8 on the scale.
During the next 75 years, other more refined versions of the scratch test were introduced including integrated microscope, stage and diamond apparatuses that applied increasing loads up to 3 grams. The material to be tested was scratched under load variants and then compared to a standard set of scratches of known value.
A more sophisticated version of this system employed a diamond mounted at the end of a tapered steel spring. The other end of the spring was connected to a balance arm with a 3 gram weight. The material being tested was moved by a hand-actuated wheel and worm gear system, on top of which sat a stage and holding fixture for the material. A fixed pressure was applied as the material was traversed, resulting in a “cut” in the material which was then measured under the microscope with the aid of filar micrometer eyepiece. A mathematical formula, inherent to the process, was then used to derive the hardness.

Pictured above: A legacy Wilson MO microhardness tester, circa 1970. Source: Wilson Hardness
Indention Tests
Later, indentation type hardness was introduced. One early form developed about 1859, was based on the load required to produce a 3.5 millimeter indent in the material. The depth was measured with a vernier scale system and the total load needed to reach the 3.5 millimeter was called the hardness. The penetrator consisted of a truncated cone that tapered from 5 millimeter at the top to 1.25 millimeter at the point. This method was mostly effective in soft materials.Another early form of indentation test involved pressing right angles geometries of the same test material into one another and measuring the width of the resulting impression. Various formats evolved from this technique during the early 1900s that likewise used “mutual” indentation of cylindrical test material with the longitudinal axis pressed at right angles to each other.
The first widely accepted and standardized indentation-hardness test was proposed by J. A. Brinell in 1900. Brinell’s interest in materials science grew during his involvement in a several Swedish iron companies and his desire to have a consistent and fast means of determining material hardness.
The Brinell hardness test, still widely used today, consists of indenting the metal surface with a 1 to 10 millimeter diameter steel or, most recently, a tungsten carbide ball at heavy loads of up to 3,000 kilograms. The resultant impression, the diameter of the indentation, is measured with a low-power microscope after removal of the load. The average of two readings of the diameter of the impression at right angles are made and mathematically calculated to a hardness value. The Brinell test essentially introduced the production phase of indentation hardness testing and opened the way for additional indentation tests that were more relevant to material types.
Around the same time as the Brinell was developing as a useful test the Scleroscope hardness tester was introduced as one of the first “non-marking” hardness-testing instruments. Albert F. Shore, who founded the Shore Instrument Manufacturing Co. in New York, and whose name is now synonymous with durometer testing, engineered the Scleroscope as an alternative hardness test.
The Scleroscope used a diamond tipped “hammer,” held within a glass-fronted tube that fell, from a height of 10 inches, onto a test specimen. The rebound of the hammer was measured on a graduated scale of Shore units, each divided into 100 parts that provide a comparison with the rebound that might be expected from hardened high-carbon steel. The hardness reading is technically a measure of the material’s elasticity.
One significant advantage of the Scleroscope was its nondestructive nature in that, unlike the other available methods of hardness testing at the time, a Scleroscope left only a slight mark on the material under test making, presumably leaving it available for use after evaluation.

Pictured above: A legacy Wilson "J" Series Rockwell tester, circa 1960s. Source: Wilson Hardness
More Efficient Testing Needs
As the 20th century progressed and endured two world wars, with the simultaneous blossoming of the industrial revolution, increased manufacturing requirements and global industrialization brought an urgent demand for more refined and efficient test methods and new techniques began to develop. Accurate, efficient forms of testing were needed in reaction to heavy manufacturing demands, structural failures and the need to design sufficient material integrity into the growing global infrastructure.As an alternative to the Brinell, the Vickers hardness test was developed in 1924 by two gentlemen, Smith and Sandland, at Vickers Ltd., a British engineering conglomerate. The test was designed in reaction to the need to have a more refined test over the material limitations than the Brinell was effective on.
The Vickers test uses the same principle as the Brinell, that of a regulated impression on the material, but instead utilized a pyramid shaped diamond rather than the Brinell ball indenter. This resulted in a more consistent and versatile hardness test.
Later, in 1939, an alternative to the Vickers test was introduced by Fredrick Knoop at the U.S. National Bureau of Standards. The Knoop test utilized a shallower, elongated format of the diamond pyramid and was designed for use under lower test forces than the Vickers hardness test, allowing for more accurate testing of brittle or thin materials. Both the Vickers and Knoop tests continue as popular hardness analysis methods today.
Although conceived as an idea in 1908 by a Viennese professor, Paul Ludwik, the Rockwell indentation test did not become of commercial importance until around 1914 when brothers Stanley and Hugh Rockwell, working from a manufacturing company in Bristol, CT, expanded on the idea of utilizing a conical diamond indention test based on displacement and applied for a patent for a Rockwell tester design. The principal criterion for this tester was to provide a quick method for determining the effects of heat treatment on steel bearing races.
One of the main strengths of the Rockwell was the small area of indentation needed. It also is much easier to use as readings are direct, without the need for calculations or secondary measurements. The patent application was approved on February 11, 1919, and later, in 1924 an improved design patent was granted.
Simultaneously, Stanley Rockwell was starting commercial production of Rockwell testers in collaboration with instrument manufacturer Charles H. Wilson in Hartford, CT. The company grew into the Wilson Mechanical Instrument Co. and became known as the premium producer of Rockwell testers. After some ownership changes through the latter 1900s, Wilson was acquired in 1993 by Instron and today has become an integral part of Instron/Illinois Tool Works. Now known as Wilson Hardness, the combined expertise of Instron/Wilson, coupled with the subsequent acquisitions of Wolpert Hardness and Reicherter Hardness, have led to the engineering and production of cutting edge hardness systems. The Rockwell test remains as one of the most efficient and widely used hardness test types in use.
Hardness testing technology remained fairly consistent throughout the mid to latter 1900s, most systems typically utilizing the dead weight method of applying the test forces. While the deadweight technique is fairly simple, reliable and was widely accepted, the method is not without some ambiguities.
Labor-intensive production, coupled with the complexities involved in a highly mechanical system depending on levers, pivots and guides led the need for further development and it became evident that other newly developed forms of force regulation used in measurement instrumentation could be applied to hardness testing as well.

Pictured above: An early version of the rebound nondestructive Sciencescope. Source: Wilson Hardness
Closed Loop Systems
With increasing demands for productivity, accuracy, user features and repeatability, closed-loop, load cell technology became a factor in hardness testing. During the 1950s, Instron pioneered the use of closed loop systems on tensile testing instruments.Closed loop systems differ from open loop (deadweight) systems in that they have a means to electronically measure the force being applied during every test and feed (or loop) the information back to the control system. The control system is designed to use the feedback to adjust the force application mechanism to apply, at an extremely accurate rate, the desired force. These systems work so well that today all electronic tensile/compression instruments use closed loop control exclusively.
With the teaming up of Instron and Wilson, the ability to adapt the closed loop control effectively to hardness testers led to the development of systems with repeatability never before realized. During the early 1990s this technology was introduced first to Rockwell testers, and later to Knoop/Vickers, as well as Brinell systems. Closed loop quickly gained momentum as a means to achieve extremely accurate and repeatable hardness test results. Today the technology is a popular and widely used format.
Now, with significant improvements in recent years in hardness testing instrumentation, computer hardware, electronics, imaging algorithms and software capabilities, the door has opened to extremely precise and reliable testing processes that provide results more quickly than ever before, often in automated fashion. These components and techniques have proven to be beneficial in raising efficiency, speed and accuracy to unparalleled levels.
During the past several years, and no doubt increasingly in the future, more traditional manual test processes have and will continue to rapidly give way to automation in every aspect of the testing process. New techniques in material preparation and handling, mount fixturing, stage movement, results interpretation and analysis, and even reporting, have now been introduced to the hardness testing industry. More and more automation technology is being integrated into many hardness systems using stage traversing and image analysis of Knoop, Vickers and Brinell indentations.
An automatic hardness system typically consists of a fully controllable tester, including an auto-rotating or revolving turret as well as actuation in the Z axis either from the head/indenter housing or from a spindle-driven system used for both applying the indent at a predetermined force as well as for automatically focusing the specimen.
Add to this a standard computer with dedicated hardness software, an automatic XY traversing motorized stage and a USB video camera, and the result is a powerful, fully automatic hardness testing system. These systems can be left alone to automatically create, measure and report on an almost unlimited number of indentation traverses. This newer technology eliminates much of the hardware that in the past caused operational challenges and cluttered workspace.
Hardness testing plays an important role in materials testing, quality control and acceptance of components. We depend on the data to verify the heat treatment, structural integrity and quality of components to determine if a material has the properties necessary for its intended use.
Through the years, establishing means of increasingly more productive and effective testing through refining traditional testing design has given way to new cutting edge methods that perform and interpret hardness tests more effectively than ever before.
The result is increased ability and dependence on letting the instrument do the work, contributing to substantial increases in throughput and consistency and continuing to making hardness tests very useful in industrial and R&D applications and in ensuring that the materials utilized in the things we use every day contribute to a well-engineered, efficient and safe world.Q