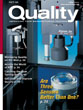
In the past decade, manufacturers improved how products are designed, manufactured, tested and certified. One of the most significant developments has been the emergence of the flexible manufacturing cell as a preferred manufacturing technique for improving the quality and reducing the cost of critical parts.
The manufacturing cell is a cluster of equipment that is ideally suited to manufacturing similar parts and part families. Because the cell is readily adaptable to different part types and can generally be managed by one or two operators, it is not dependent on high volume runs for its economic justification. Whether the cell is making thousands of one part type, or just a few, the number of parts in each run is of little consequence, as long as the cell is always making something, and doing it to within tolerance.
Flexible manufacturing equipment in a cell requires flexible, cell-capable measurement systems that can be used to adjust and verify setup, monitor relatively short-lived manufacturing processes and get the most out of increasingly tight part tolerance budgets. Perhaps the most flexible of all high-end measurement approaches is the use of multisensor coordinate measuring machines (CMMs) that incorporate touch trigger probes, vision metrology systems (VMS) and laser technology.
Most people in manufacturing have an intuitive sense that three sensors are better than one. They also have understandable trepidations about how well multisensors can be integrated into a single precision measurement system, how well operators will assimilate knowledge required to operate different types of probes, and how much more this technology might cost.
Probing the technologies
Although the multisensor measuring systems of the 21st century have their roots in other CMM and VMS technologies, their designers are ultimately judged by how well they combine and balance these technologies to create a machine with significantly greater capability than any of the parts. The technologies include CMM touch probes, VMS camera optics and captured picture conversion, laser-measuring devices, and software and machine design.
The touch probe is by far the most researched technology within the metrology community and provides enhanced capabilities within the context of multisensor systems. Touch probes run the gamut in terms of sizes and applications. For example, a spherical ruby type probe can range from 0.3 millimeter up to around 10 millimeters in diameter, as well as cylindrical and conical probes.
Touch probes are best used for measuring 3-D geometrical features that require a surface model calculation for fit, size and location. Although touch probe technology is still advancing, its general use requirements are the most widely understood. Critical issues for touch probes are how much staging the parts require and, for deep bore measurements, the reach of the probe stylus.
In contrast to touch probes, which must move in space and time from point to point along the part's surface, the cameras used on vision metrology systems require no surface contact and can capture thousands of data points almost instantaneously. They can reach down in areas or constructions where conventional probes do not fit such as small angles and small radii.
End users also have choices when it comes to cameras, and each of these camera types have its own set of advantages and disadvantages. For example, zoom cell cameras generally provide greater magnification ranges, but in doing so, tend to sacrifice accurate repositioning. This problem is the result of two types of errors inherent to all mechanical zoom optics, "par centricity" and "parfocality." Par centricity deals with how well an image maintains its position in the field of view, and parfocality deals with how well the zoom lens maintains acuity of focus -- particularly on the edges -- as the zoom cell moves to various positions from 0% to 100% magnification.
Because the amount of error in effect at any given position is not proportional to the percentage of magnification, calibration of the zoom cell is necessary to ensure 3-D accuracy. The industry is currently working on a set of standards that manufacturers must follow when calibrating their zoom cells. When these standards become widely used, there will be a closer correlation of data produced by VMS models employing zoom cells. Currently, a standards committee is actively working to define the standards.
Choices
Other vision metrology systems have fixed optics systems like those used in microscopes. These have significantly greater repositioning accuracy to limit par centricity and parfocality errors. The challenge in these systems may be in a lack of field-of-view or depth-of-view measurements. Some recent camera designs implement both fixed optics and zoom capabilities to achieve a greater magnification range without sacrificing positioning accuracy. The cameras can alternate between measuring small features that fit within a field of view, or about 1 mm2, to large features of about 8 mm2 to 1,000 mm2 within a field of view in a matter of seconds.
Captured picture conversion is another consideration. An image is captured in the camera when the lens assembly directs it onto the pixel receptors of a charge-coupled device (CCD). The analog signal is then converted in the camera to a digital signal that is sent to a video board for analysis. The camera is the part of the vision system that converts the image.
There are three types of cameras that may be employed by multisensor systems:
- Black and White. Relatively inexpensive black and white cameras provide the least contrast change and, therefore, deliver results that are generally less accurate and repeatable than other types of cameras, barring any specialized customization such as cameras that are used in in-line character recognition systems. Black and white cameras can be cost-effective for 2-D parts with sharp edge contrast and low accuracy requirements.
- Color. Of course, if colors need to be differentiated to capture a viable image for analysis, a black and white camera will not do the job. Color cameras are the most expensive choice, and are also limited because the requisite filtering prior to the analog signal capture can block up to 50% of the light transmitted through a lens assembly. Another problem with the color camera is its sensitivity to certain light spectra. For example, excessive red can bloom, which means that the red can overflow into adjacent CCD array cells to overpower the other color spectra, causing a blurry or ghostly image. For this reason, color cameras are generally considered the least useful for vision and metrology.
Along with the black and white camera, the color camera is generally used in Object Character Recognition (OCR) and Object Character Verification (OCV) applications. These provide visual confirmation that the components are in the correct position.
- Gray Scale. Most vision and multisensor systems rely primarily on gray-scale camera designs. These allow for the analysis of 256 shades of gray for the accurate and repeatable determination of edge and surface variations for many types of parts and materials. If some degree of color recognition is needed, all is not lost. Camera technologies are under development that would be better capable of colorizing gray-scale images and these should be available within the next five years.
Laser options
Multisensor systems frequently employ lasers to supplement video technology for extremely accurate and rapid capture of noncontact measurement data. Lasers have been used since the early 1980s for such purposes as collecting reverse engineering data from models. Unfortunately, the common use of triangulation, which is the bouncing of the laser signal at an angle of incidence of approximately 45 degrees, has limited the usefulness in capturing data from complex parts with deep features. This problem was attenuated by the introduction of a technology that permits low incidence angle lasers that can work with angles that are less than 30 degrees.
Even more promising are the on-axis through the lens (TTL) laser systems that follow the optical path and require no physical offset values when changing between camera and laser measurement. On-axis TTL laser systems have proved to be more flexible and repeatable than triangulation laser probes and the system shortens the time required to switch between video and laser measurement because of the close correlation between laser and vision system alignment.
Machine design
Many original metrology equipment manufacturers have recognized the need for multisensor capabilities and some have answered that need by adapting existing single sensor machines to accept additional sensors. Users who have purchased this type of system have frequently been disappointed with the performance of the added sensors. This is not surprising. All of the considerations that make for a sound CMM design must be incorporated into a multisensor system and more. For example, a robust machine with temperature stable materials is a good starting point. Good vibration damping is also an extremely important feature for capturing measurement data with a camera.
Perhaps the most important machine design requirement is the grouping of sensors. They should be grouped as closely as possible so that minimal physical adjustment and compensation are required when changing from one sensor to the next. This consideration has a profound impact on the precision, throughput and flexibility attainable with the multisensor system.
In addition to integrating three types of sensors into a single automated measurement system, good multisensor design must also encompass software integration so that the user can readily create programs that flow effortlessly from one sensing technique to another. This is facilitated by the use of powerful, intuitive, CAD-based, off-line programming capabilities.
All manufacturers say their software is user-friendly, but ultimately the user must be the judge. Meeting part programming requirements for the average part may be good enough for a single sensor system. But it is not good enough for taking full advantage of multisensor measurement capabilities to meet monitoring and control requirements for flexible manufacturing cells.
Could it be?
A single flexible tool that can be used in a manufacturing cell to measure all the critical features in an entire family of parts with one setup per part almost sounds too good to be true. Before acquiring a multisensor system, prospective users have to ask themselves, "Is this really a viable technology for my manufacturing cell application, or is it just an expensive Swiss Army Knife that does a little bit of everything, but nothing all that well?" The answer to that question breaks down to just a handful of issues:
- Precision. There will almost always be times when critical dimensions must be measured with extreme precision. The weakest link in the multisensor system's precision measurement chain has been optics. Today's two-stage fixed and zoom optics can provide submicron resolution over a wide field of vision. Even higher precision optical solutions will arrive within the next three to five years.
- Integration. High-level integration of a good multisensor system means that programming the system to change from one sensor to another is no more difficult -- perhaps even less difficult -- than changing probes with a direct computer controlled (DCC) or computer numeric controlled (CNC) CMM. A good multisensor system will provide seamless measurement results with no significant loss in the error budget from positioning when changing from one sensor to another. In fact, with a correctly configured multisensor machine, overall accuracy can be significantly better than that achieved when setting up the same part on multiple measurement systems.
Significant time savings can also be achieved. For example, in one application involving measurements of multiple features on a medical part, the use of a multisensor system reduced part handling, setup and inspection time dramatically as compared to using multiple systems to do the task. In comparison tests using a multisensor machine, it took three minutes to set up the medical part for touch probe and laser measurement, and then 12 seconds to run the measurement program and transmit the data to an offsite location. On a more conventional machine, setup for touch sensors alone took between five and eight minutes. Relocation and setup on a vision system took about the same amount of time, plus an additional three to five minutes to verify that the datum setup correlated with the CMM.
In these tests, the multisensor CMM measured all of the features in less time than it took to do one function with multiple machines. One reason it can do this is the synergy that multisensor machines can offer. For example, the definition of a plane with the laser focus points provides rapid response within milliseconds, whereas a touch program will require a couple of seconds, if not a full minute, for positioning.
- Ease of Use. There is no question that users who are versed in only one measurement technology such as conventional CMMs will have to learn new skills. One of the biggest areas for most will be learning how to illuminate parts effectively to optimize data capture by video technology. The good news is that the basic multisensor operation can still be taught in a standard, relatively short, training course. During the learning stages, sensor wizards in the software can help users get past their initial unfamiliarity with different technologies.
- State of Development. A major benefit of having multisensor technology in a cell is being able to meet future measurement needs. It's a "center court" technology, which means that it matches well with the inherent flexibility of the manufacturing-cell environment. However, potential users are concerned that new developments, particularly new sensor technology, could rapidly outdate today's multisensor systems. It is true that some interesting developments, such as ultra-high optics, are on the horizon. However, if the user invests in a true multisensor system, as opposed to one in which additional sensor capabilities are added as an afterthought, he or she need not fear that the system will become outdated. Well-designed equipment and software will serve as a stable platform to accommodate new sensor technologies as they are developed.
- Relative Cost. Multisensor measurement systems cost somewhat more than single sensor equipment, but they also do a lot more. The contribution they make to improve quality and throughput in a manufacturing cell should more than offset the additional cost increment.
While the market for multisensor systems is still in the early development stages, many different systems and sizes are already available. They range in cost from about $70,000 to about $500,000. Being able to choose the right-sized system for the application reduces cost impact. It is also possible to buy the basic system with only one or two sensors and add others as they are needed.
After addressing these cost concerns, the bottom line is simple: Multisensor measurement is a promising technology with a bright future. If you are developing a manufacturing cell that would benefit from this style of measurement, the technology is far enough along that there is no reason why you should not begin investigating it today.
TECH TIPS
- Multisensor measuring equipment is flexible enough to be used in a manufacturing work cell. Multisensor equipment includes touch probe, vision metrology systems and laser systems.
- Software must allow users to readily create programs that flow effortlessly from one sensing technique to another.
- Sensors should be grouped as closely as possible so that minimal physical adjustment and compensation are required when changing from one sensor to the next. There should be no significant error budget loss from positioning when changing from one sensor to another.
- Fixed and zoom optics can provide submicron resolution over a wide field of vision.