2017 was a transformative year for the materials testing industry.
First, Element acquired Exova, creating a combined global testing organization of more than 6,200 employees that now operates as Element Materials Technology; the new Element Group will include more than 200 laboratories across more than 30 countries.
Element also acquired cardiovascular testing expert Medical Device Testing Services (MDT), a move that legally merged MDT’s Minnetonka, MN, laboratory with Element’s own global center for medical device testing, Element Cincinnati.
Meanwhile, U.S.-based 3D printing startups and research labs made progress in developing hybrid polymers for additively manufactured medical devices that are tough, durable, biocompatible and, of course, 3D-printable.
The road to market for these new materials and devices is long, however, as the U.S. Food and Drug Administration (FDA) and other regulatory bodies like ISO and ASTM must validate the devices’ strength, durability, and safety, often through independent testers like Element, and approve them for patients’ use.
And yet, the road is clearing. In December, the FDA issued the guidance document “Technical Considerations for Additive Manufactured Medical Devices,” which represents a huge step forward for the administration insofar as openness to innovation in the field.
“3D printing is certain to alter the daily practice of medicine where patients will be treated with medical products manufactured specifically for them,” FDA Commissioner Scott Gottlieb, M.D., said in a statement. “The FDA has an important mission to help advance these efforts while also protecting patients who depend on medical products to be safe and effective.”
A shift in focus
In 1988, the company 3D Systems introduced the first commercially available 3D printer, the SLA-250. Thirty years later, 3D printing materials, more than the printers themselves, seem to be driving applications in healthcare
Katie Weimer, vice president of medical devices for Denver-based 3D Systems Healthcare, has seen this firsthand. In a 2017 interview with Disruptive, a website focused on the additive manufacturing industry, Weimer discussed the industry’s continued drive to develop new materials that are biomimetic.
“We want anatomical models of bone to act more like the hard and soft tissues in the human body,” she said. “For example, we are working to print heart models that simulate real heart tissue so a surgeon can simulate the surgery with cutting and suturing and the model behaves just like a real heart.”
Weimer also pointed to 3D Systems’ Figure 4 platform for high-speed, production-ready plastics as another advancement. Figure 4 offers multi-mode polymerization, or manufacturing parts in hybrid materials that, according to Weimer, are tougher, more durable, more biocompatible, and more resistant to temperature and deflection than the materials already approved by the FDA.
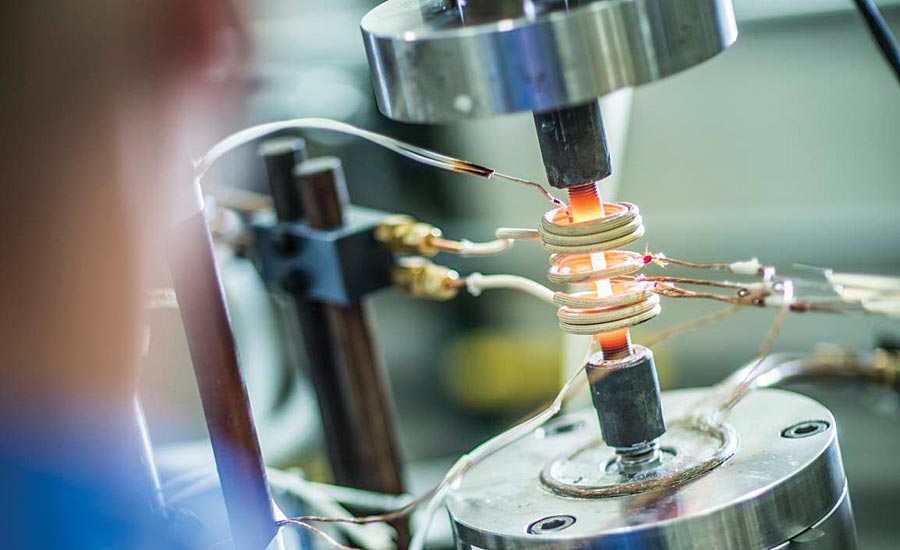
Experts at Element Cincinnati’s high-capacity Materials Testing Lab engage in mechanical testing, metallurgical analysis, material characterization, and microbiological evaluation of medical devices. Source: Element Materials Technology
And the materials are…
Though still in development, new hybrid materials—and new ways of making them—hold promise for a future in which medical devices will be more cost-effective, readily available, and tailored to fit individual patients’ needs.
Carbon Inc., a technology company and manufacturer based in Redwood City, CA, uses Continuous Liquid Interface Production (CLIP) to create 3D printable, biocompatible, and high-temperature resins that could be used in medical devices.
The process begins with a pool of liquid photopolymer resin and a beam of ultraviolet light, which causes the liquid to solidify. Once UV polymerization has been achieved, the second step, applied heat, turns on a latent chemistry in the polymer to create new hybrids. These include more durable and biocompatible resins, elastomers based on silicones, and polyurethanes that range in properties from nylons to thermoplastic urethanes.
In 2017, researchers at Children’s Minnesota Hospital developed a new airway stent for preclinical testing, enabled by Carbon’s M Series printers and SIL 30 material: a silicone urethane that is the first additive material to offer a unique combination of biocompatibility, low durometer, and tear-resistance.
Carbon’s partnership with healthcare giant Johnson & Johnson to create surgical tools, announced in 2016, also paves the way for Carbon’s technology and materials to continue advancing in this realm.
Meanwhile, university researchers are using CLIP-like methodology to create their own biocompatible, 3D printed implants that can be customized for a patient’s specific anatomy.
In 2016, Northwestern University professors Guillermo Ameer and Cheng Sun teamed up to 3D print customized vascular stents. Using Sun’s microCLIP 3D printing technique and a citric-acid based polymer previously developed in Ameer’s lab, they found that the resulting stent was flexible, biodegradable, and contained inherent antioxidant properties.
Their research, published online in the journal Advanced Materials Technologies and in an article on Northwestern’s website, is supported by the American Heart Association.
Other promising materials recently developed by Northwestern researchers—this time in the university’s Shah Tissue Engineering and Additive Manufacturing Lab—involve live cells.
In 2017, as part of a study by Northwestern’s McCormick School of Engineering and Feinberg School of Medicine, Shah Lab’s hydrogel PEGX technology platform was used to print bioprosethetic ovaries from a biological hydrogel. When an infertile mouse’s ovary was removed and replaced by the hydrogel ovary, the mouse ovulated, gave birth to a healthy litter of pups, and nursed them afterward.
Ramille Shah, assistant professor of materials science and engineering at McCormick and of surgery at Feinberg (and the lab’s namesake), spoke to Northwestern News, the university’s online news platform, when the research was published in the journal Nature Communications last May.
“Most hydrogels are very weak, since they’re made up of mostly water, and will often collapse on themselves,” Shah said. “But we found a gelatin temperature that allows it to be self-supporting, not collapse, and lead to building multiple layers. No one else has been able to print gelatin with such well-defined and self-supported geometry.”
Dimension Inx, a spin-off company of Shah Lab, is moving forward with another technology the lab developed, called 3D painting. Though not yet approved by the FDA, material solutions such as hyperelastic bone and 3D-graphene are potential game-changers for the healthcare industry.
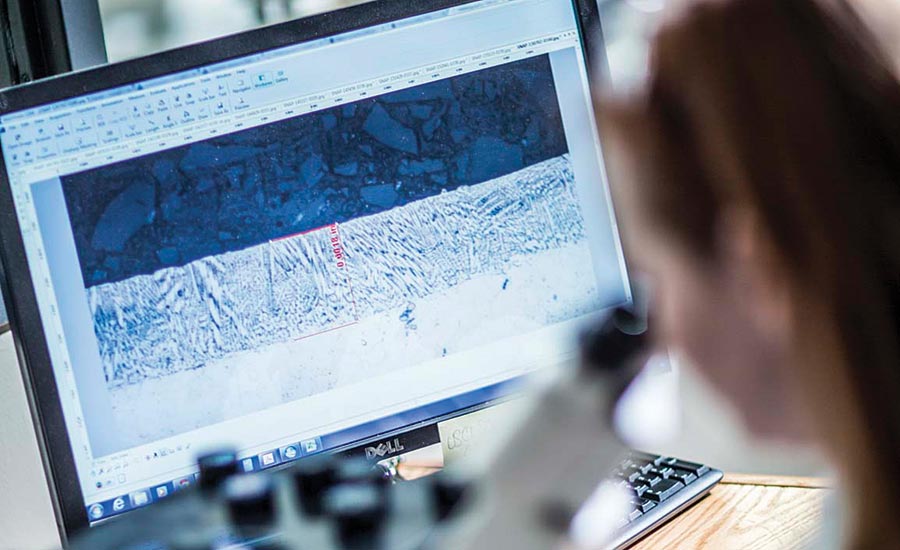
The distinction of 3D painting lies in the flexibility of application. According to the website description provided by the Dimension X team—which includes Shah, co-founder and chief science officer; Adam Jakus, co-founder and CTO and John Hartner, CEO 3D paints are capable of being “co-3D-printed with each other to create multi-material structures and products, or mixed prior to use to create hybrid 3D-Paints with unique properties.”
Hyperelastic bone, for example, is a medical-grade, bioactive ceramic with “the potential to repair and regenerate bone without the need to add additional cells or growth factors,” i.e. stem cells.
3D-graphene, a majority graphene composite that Dimension Inx describes as “highly electrically conductive” and “easy to 3D print into 2D or 3D forms,” also doesn’t require the help of stem cells to grow nerve and muscle-like cells within the body.
Challenges ahead
Maciej Jakucki, operations manager at Element Materials Technology, spoke to Quality about some of the issues that additive manufacturing and technology startups face when bringing a new medical device to market, and how Element can help.
“We can offer initial consulting, validation, and troubleshooting with processes, all the way up through part manufacture and initial feasibility testing,” Jakucki says. “For us, it’s been really transformative, because we can step into the medical device industry as a consultant, as an expert, and help them in all aspects of the process.”
To evaluate whether a 3D printed product is as strong, safe, and clean as a traditionally manufactured one, Jakucki says that companies who lack the internal resources for testing must find accredited labs to do so, or risk increasing time to market by getting mired in regulatory pushback.
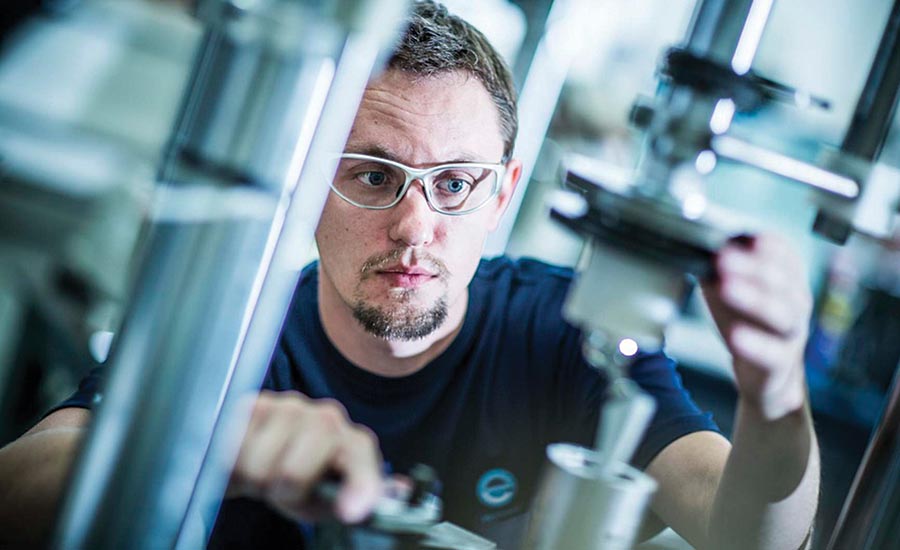
“Most test labs are accredited by ISO 17025, and some have internal quality systems as well,” Jakucki says. “So it’s critical to make sure you’re maintaining a level of quality, because that will help your submissions get through quickly.”
And while Jakucki says he hasn’t seen a lot of new materials for medical devices come through over the last 30 years (“Realistically, you’re limited by biocompatibility”), he says he is impressed by additive manufacturing endeavors to replicate and even invent new structures to help facilitate osseointegration.
He uses porous coating as an example.
“Traditionally, you’d take a medical implant, design it, and then spray it or blast it with a particular coating. Now, you can just print it,” Jakucki says. “You can control the porosity and strength levels, and try to optimize bone ingrowth—which has implications on recovery times and durability, how long implants will stay engaged within the body.”
“There’s a lot of discussion in the industry for how to evaluate things like porous coatings and new implants,” he continues. “Ultimately, the product needs to be evaluated for its strength and mechanical integrity as a form of function, and not necessarily material. The material is a part of that for sure, but it has to function within the design that you create.”