In 2010 the ASTM F42 Committee on Additive Manufacturing (AM) created the terminology for an industry which up to then was referred to as rapid prototyping (RP). Parts made using the various rapid prototyping technologies were often used as design concept models, fit check mock-ups and function test articles. Short-run end use part applications developed where RP parts were used as patterns for the cast urethane industry, producing polymer parts with appropriate mechanical properties. Dimensional tolerances and surface finish were important, but other quality metrics were often not required. The facilities that manufactured these parts seldom held a QMS and were generally called service bureaus.
One of the earliest examples of production using RP, in 2001, were the electronics cooling ducts for the F/A-18 produced by Boeing spin-off On Demand Manufacturing (ODM), based in Camarillo, CA. These parts were produced out of nylon 11 using the technology powder bed fusion, known by trade name selective laser sintering. As a producer of aerospace production parts, it was a requirement for ODM to implement powder bed fusion into their AS9100 QMS and the era of contract manufacturing using AM began.
Because of the layer-wise method of producing parts by AM, the process has been historically slow compared to conventional manufacturing methods. With the AM machines being capital intensive and the process relatively slow, the per piece cost point has been high and only the high value markets such as aviation, space and medical implants could afford to adopt the technology. These are highly regulated industries where the consequence of part failure can lead to millions of dollars in damage and loss of life. Today, it is common for facilities that integrate AM into their facility to have a high-level QMS, such as ISO9001, AS9100, ISO13485, Nadcap accreditation and ASTM AMQ. Integrating AM processes with a QMS has proven to be significantly challenging.
There are two main reasons why the implementation of AM processes with a QMS have been difficult. First, tribal knowledge necessary to process “good” parts has been primarily held within a relatively few experts at small businesses that typically have a limited quality team or no designated quality team at all. It is routine for key employees to be responsible for several sections of the QMS and as a result, quality escapes were common. The second reason why implementation of the AM process with QMS has been difficult is because AM is generally a special process where the part attributes and the part form arrive simultaneously. Slight changes in process can lead to significant changes in quality. The heroes of the service bureaus were knob turners who could adjust the speeds and feeds on the fly to produce good-looking parts. Unfortunately, this is counter to the fixed process necessary to achieve consistent part quality.
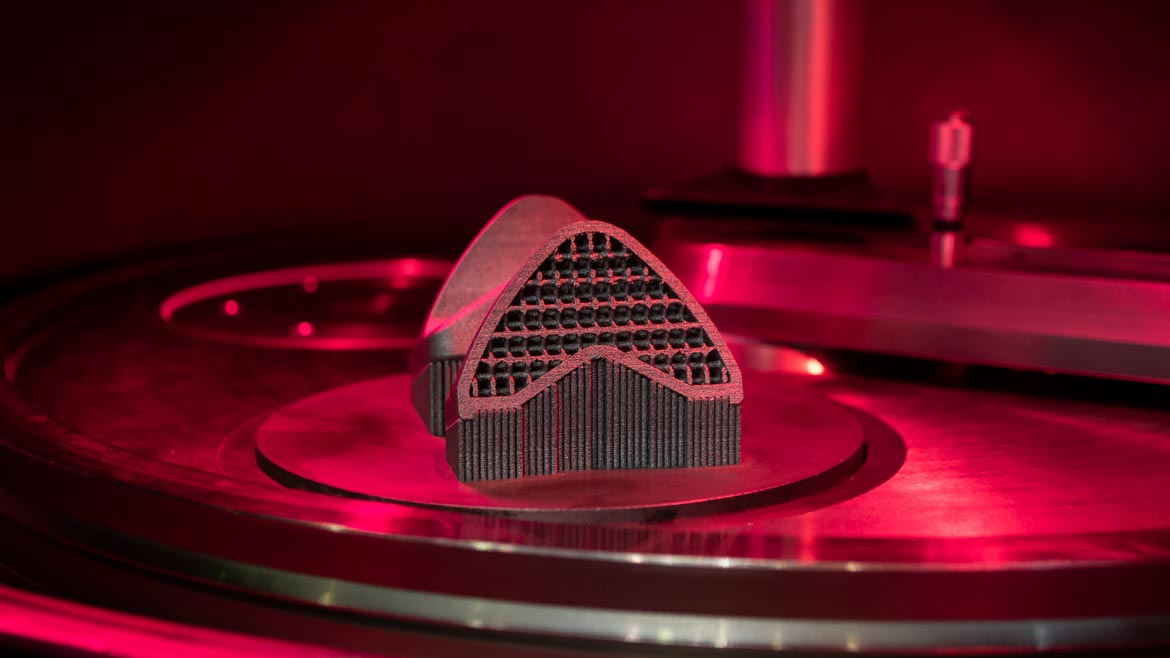
Image Source: Marina_Skoropadskaya / iStock / Getty Images Plus via Getty Images.
As of today, FDA cleared over 200 medical devices falling under different device types. The majority of them are fracture critical orthopedic implants. Thousands of such medical implants are produced annually using the powder bed fusion process and titanium alloys. If someone today has a spinal fusion surgery, chances are the spinal cage that is inserted between vertebrae was produced by powder bed fusion, and additively manufactured hip replacements are becoming common. The drive behind this evolution is an increase in positive patient outcomes due to design freedom and process industrialization.
AM produced parts are finding their way into many satellite applications where there are limited fatigue requirements as the satellite rockets into the weightless environment. There are also many examples of AM being used in aerospace, however, these are typically non-structural parts where dynamic part property requirements are limited. The exception to the non-structural generalization are the rocket propulsion systems that are being designed and produced with metal AM. The space-race the world is currently experiencing would not be possible without AM reducing schedules from years to weeks, using designs that are more powerful and compact than anything possible in the past. We are also seeing parts produced with AM using refractory alloys that would not be possible using conventional manufacturing technology. These materials withstand extremely high temperatures and will enable novel designs in rocket engine thrusters and hypersonic flight. More structural applications and high cycle fatigue applications are in development and test stage and in the next five years some surprising aerospace applications are poised to be publicly released in turbine engines and airframes using nickel, aluminum and titanium alloys.
To facilitate widespread adoption of AM it will be necessary for material property data to be shared among the design community at large instead by being held by a few large companies. While this sounds simple, it is a complex problem, primarily due to cost. Understanding a material’s static and dynamic properties across the temperature spectrum can cost more than $500,000 per material and can eclipse $1M on exotic materials. Once this cost is factored into a part or part family business case, the use of AM is often cost prohibitive. Also, there is not industry agreement as to what AM process quality control conditions are necessary prior to producing the test specimens used to create the material property data. The material data sets produced to date have been through propriety material and process specifications by large corporations that value the data as a competitive advantage. For widespread adoption of AM, both the machine equivalency certifications and the material property data must be democratized through shared data and industry consensus standards.
AM experts generally agree that for most powder bed fusion metals and often directed energy deposition material can have properties that exceed cast materials. Additionally, there is growing consensus that qualified PBF processes produce materials with fewer and less detrimental anomalies than castings. In fact, if the casting process was a recent development, AM metals would be the preferred fabrication process (not accounting cost). However, the acceptance of AM metals lags far behind cast materials because we have centuries of experience with castings and nondestructive evaluation (NDE) techniques that classify and grade casting according to age tested standards. The other benefit of a centuries old manufacturing technique is that over time the use of castings has self-classified into applications and part families that are appropriate for the technique. By contrast, AM metals have yet to develop these quality metrics largely identified in the MMPDS. However, both the NDE techniques and part classification methods for AM are about to be resolved in ASTM F42 Committee on Additive Manufacturing.
There are two Work Items under development in the Applications Subcommittee F42.07 that once published should provide the foundation for metal PBF to be widely adopted. Work Item WK70164 “Additive Manufacturing – Standard Specification for Assigning Part Classifications for Additive Manufactured Parts Used in Aviation”, provides a framework for making a part classification based on the consequence of failure. Metal AM parts will be given an A, B, C or D classification that will allow for material and process specifications to call out appropriate requirements for process controls, post process thermal treatments and NDE with an eye toward affordability. A rigorous decision matrix will prevent over specifying quality controls that are unnecessary for the application. Alternately, WK70164 will ensure parts with a high consequence of failure are treated with the highest level of quality scrutiny.
WK75329 “Additive Manufacturing – Standard Practice for Nondestructive Testing (NDT), Part Quality and Acceptability Levels for Additively Manufactured Aerospace Parts processed by Metal Powder Bed Fusion” establishes the specific to AM requirements for parts inspected by NDT methods. Process anomalies such as keyhole porosity, lack of fusion porosity, and gas porosity will have established maximum dimensions and inspection levels. The combination of WK70164 and WK75329 should provide the confidence necessary to allow for widespread adoption of metal AM and ensure part quality meets all requirements.
Additive manufacturing has the capability to become a common part processing technique that will be adopted in highly regulated industries first and then more broadly as the cost per part decreases. Sharing material property data among the design community will accelerate the adoption of AM as qualification costs are shared and barriers to entry are lowered. In order to assist the AM community in the adoption of additive technologies, the ASTM COE was created to provide research that leads to consensus standards and to provide a number of personnel trainings that range from AM quality assurance to facility safety protocols. Two new standards currently under development in the ASTM F42 Committee will provide adequate quality assurance to cognizant engineering organizations that commit to AM to the point where there is no other manufacturing alternative. ASTM Advisory Services can help achieve these goals. Then, we will see the true goodness of AM. For more information on the ASTM Center of Excellence, please visit https://amcoe.org.