Though buzzier than ever in 2019, 3D printing has existed for at least 35 years, beginning with the invention of stereolithography in 1984 and accelerating with the development of fused deposition modeling in 1988. More recently, however, additive manufacturing for series production, paired with an uptick in lower-cost metal 3D printers, has elevated the industrial 3D printing industry to previously unreachable heights.
Beyond being used in the development phase to create functional prototypes and molds, 3D printing technologies are now highly desirable in the aerospace and defense, aviation, and automotive industries for series part production. Increasingly, OEMs in these sectors are learning that additive parts can be more complex in their designs yet more efficient to produce. They’ve also discovered that they can use an additive process to improve a part’s performance and reduce its weight.
Nevertheless, questions and concerns about quality remain a sticking point, particularly for safety-critical parts like 3D printed components for jet engines. Customers of 3D printing machine and process vendors want to know, justifiably, that the quality of the additive parts, and of the process and system used to be produced them, will meet their requirements.
PROCESS, SYSTEM, AND MATERIAL
EOS, a global company that specializes in producing additive manufacturing machines and industrial solutions, is known for its direct metal laser sintering (DMLS) and selective laser sintering (SLS) technologies. For both, the company has offered what they call “a holistic approach” to quality assurance, encompassing “the three technological elements of additive manufacturing that have a direct influence on the quality of an additively manufactured component.”
These elements are process, system, and material. The joint development and mutual adjustment of these three elements, according to EOS, establishes the conditions necessary for the best possible part properties.
“The reason we take the approach to standardizing the process, system, and material together is that it eliminates variables in the overall process,” says Caleb Ferrell, general manager of Advanced Laser Materials (ALM) in Temple, Texas; ALM is a wholly owned subsidiary of EOS Group. “When we’re talking about making repeatable parts with AM technology, this type of approach is essential, especially for critical parts in aerospace that are used in the engine or turbine.”
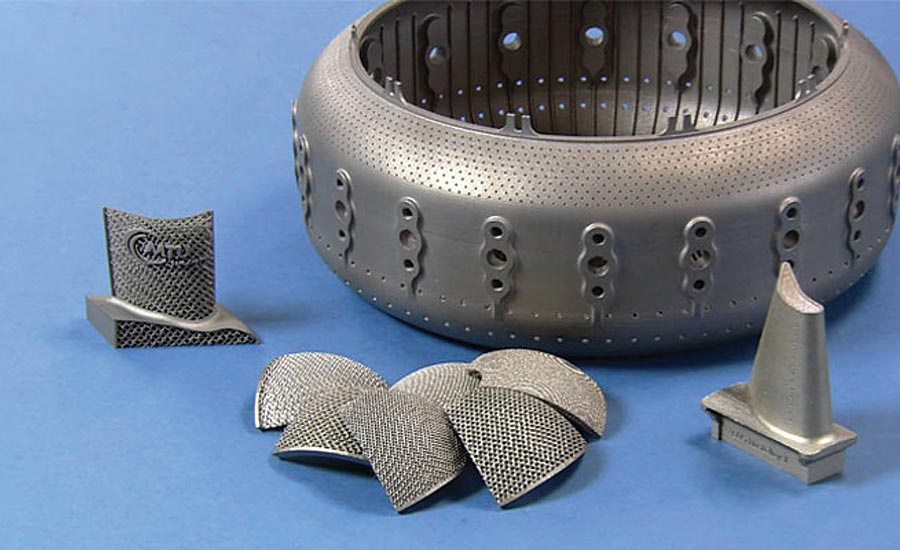
A collection of 3D printed parts for MTU Aero Engines. Source: EOS
Process
When talking about quality control measures in the process, “we mean build parameters, build layout, and part files,” Ferrell says. Generally, EOS recommends checking and validating all process parameters—laser power, layer thickness, etc.—that are necessary for achieving certain component characteristics and that are based on decades of experience and comprehensive tests.
Required product characteristics can be achieved through the optimum combination of individual parameters, and data sheets that provide a detailed description of the material characteristics that can be gained.
System
The system “means calibration, maintenance, and build-to-build variance,” Ferrell continues. Reliably meeting manufacturing standards is not so difficult if one uses laser sintering technology, for example, which is certified to ISO 9001 and has been employed by EOS for more than 20 years.
Additionally, EOS machine acceptance tests incorporate comprehensive checks of all system components and of defined reference parts. Test criteria include mechanical properties, tensile strength, elongation at break, surface quality, and part density, with DIN and ISO standards applied.
Material
For materials, “we’re looking at supplier quality, recyclability ratio, and environmental controls,” Ferrell explains. At EOS, guaranteed and consistent quality of every material batch starts with examining each batch for its correct chemical characteristics, as well as checking for consistent grain size distribution.
EOS guarantees consistent and reliable quality in every material batch with a multi-dimensional approach to quality management. The company’s Oy branch in Turku, Finland, for instance, focuses on the development, qualification, and quality assurance of metal materials, and is certified to ISO 9001 and ISO 13485. Here, density cubes and tensile bars are manufactured and analyzed according to fixed criteria under standardized conditions. A mill test certificate also is included with each delivery.
Each of the three technological areas delineated by EOS—process, system, and material—is equally critical, Ferrell says. “But, just like making a cake, it starts with the ingredients you first put in. If you use a poor quality material, you will get a poor quality part.”
THE ROLE OF QUALITY IN AM FOR SERIES PRODUCTION
Chuck Hull, the co-founder and CTO of the additive manufacturing company 3D Systems, pioneered stereolithography (SLA) technology in the mid-1980s. Today, 3D Systems produces metal, plastic, and dental 3D printers, as well as materials, software, and end-to-end manufacturing solutions that feature SLA, SLS, direct metal printing (DMP), and other 3D technologies.
In 2018, the company launched its DMP Flex 350, Factory 350, and Factory 500 systems. The Flex 350 is a high-throughput, high-repeatability metal 3D printer that generates precision quality parts. The Factory 350 platform is an upgrade to the Flex 350, providing integrated powder management. The Factory 500, developed in partnership with GF Machining Solutions, is a set of five function-specific modules for companies looking to scale their metal AM production.
Along with other major players like EOS, Stratasys, Renishaw, and GE Additive, 3D Systems has positioned quality as a top focus for AM in production. Scott Green, director of project management at 3D Systems, contends that inspection for additively manufactured parts is in many ways similar to inspection for parts produced by other technologies, but it depends on the user’s role.
“In early-stage technology vetting, there will be significant rigor around vetting a specific technology or machine vendor,” says Green. This is because the part integrity, quality, and long-term durability of an additive part must be measured, and also verified as dimensionally acceptable. Studies will take place using traditionally manufactured parts and materials as benchmarks, in addition to destructive and nondestructive testing, before the technology can even enter a manufacturing environment.
Green also predicts an increase in non-contact optical scanning and CT scanning, which machine vendors will increasingly offer on their machines. Build monitoring will likely gain the most mindshare, he adds, as it provides a significant layer of quality control and monitoring that helps brings safety and comfort to the implementation of AM technology in production.
This is where Industry 4.0 comes in. “People tend to trust the process in the early stages if they can see, predict, or understand problems,” Green says. After all, additive is working against decades of computer-driven and traditional manufacturing processes that are well-known and trusted, i.e. methods from Industry 3.0.
On the design and manufacturing engineering side—particularly during product ramp-up and working toward first article inspection—Green forecasts a rise in root cause analysis. Considering that the technology and the materials have been vetted, the customer’s main focus will be to produce the highest-quality and most dimensionally compliant part, created with the most stable and predictable process possible.
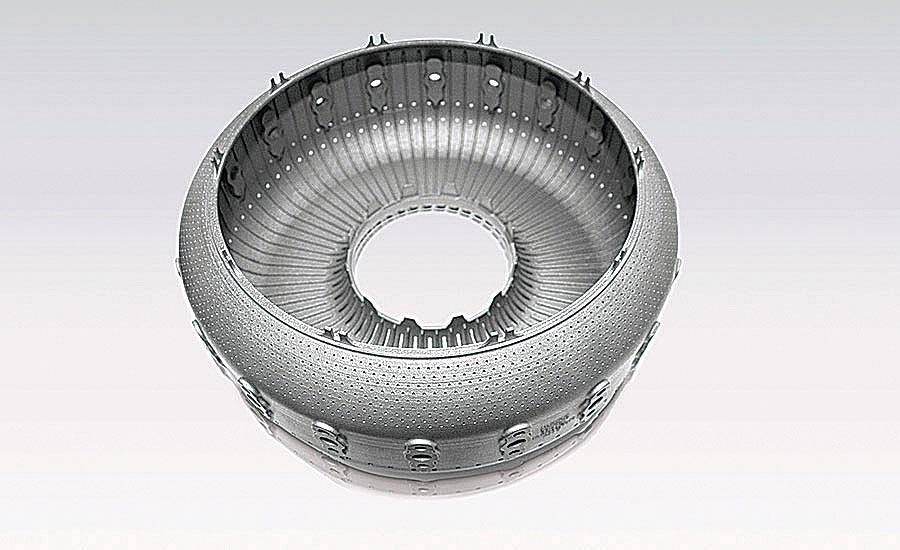
A nickel alloy jet engine combustor made with DMLS. Source: EOS
“In this case, definitely there is some space for innovation and new software tools that diverge from traditional manufacturing inspection,” Green continues. “It’s going to be relatively painful to do root cause analysis on additive parts if you have to use software tools made for traditional manufacturing. Here you will see some innovation in software and printers to better understand things like warp, quality of prismatic features, localized surface deviations, and repeatability—and also to trace those back to actionable information, which can drive some resolution or change in the printing process and ultimately make better parts.”
In production stages, Green thinks that “very little might change for the real ‘inspector’ of high-volume production parts.” The general customer requirement will be the same, “Did you deliver a part which meets my requirements?” In this scenario, the customer’s requirements and GD&T on the part, plus geometric controls, will come from traditional manufacturing methods—“and these requirements really revolve around measuring the accuracy or compliance of a part with respect to its actual function,” Green explains. At the same time, “we should also be watching the relevant standards bodies to see if they make any accommodation for additive in the GD&T definitions.” So far, Green says he sees “no indication” of this happening soon.
THE FUTURE OF AM IS BRIGHT, IF SOMEWHAT UNCERTAIN
Ferrell also views regularity hurdles as a barrier to continued metal AM adoption, but maintains that they’re certainly clearable. “Quality requirements for additive manufacturing have yet to be fully standardized, which creates added difficulty for quality professionals when they first start to analyze whether an AM part quality is good or bad,” he explains. “The key to ensuring a high level of quality—especially in industries with stringent quality measures such as aerospace, medical devices, energy, and automotive—is to partner with customers to write specifications and design allowables for the additive process.”
“Speaking strictly on quality, additive manufacturing is ready for serial production,” Ferrell continues. “EOS has high adoption rates with customers in those tough, quality-stringent industries, from aerospace to medical device manufacturing. The next step is figuring out how to scale for production rates. The world has high demand for mass customization solutions, such as athletic wear or replacement parts for a vehicle, and additive manufacturing is the perfect fit. As an industry, we must find more solutions for customers that are innovative and economical.”
At 3D Systems, Green has a similar mindset. The generally high cost of high-precision metal AM production has impeded the technology from scaling, he says, but it’s also led to lower-cost metal AM machines gaining traction. “You may also see additive being used as a tool, or surrogate or placeholder, for another downstream process,” Green attests. “You might not be pulling a print right off a DMP 350 machine from 3D Systems, finishing it, and throwing it in your Ford, but a Tier 2 or 3 supplier of castings for Ford is probably going to adopt additive as a method to be more efficient in their ability to serve their customers as a supplier.”
Moreover, new technologies driven by predictive analytics are progressing at a rapid clip. “The industry trend is: cameras are cheap, computers are cheap, cloud is available, and you’ve got plenty of opportunities to take and measure data from your machines,” Green notes. “New additive technology is going to be very different than we’ve had before, because now it’s very easy for us to harvest data from a printer, learn from that data, and reapply it to the process to make better printers and better components. It’s now easy for us to measure what’s happening inside the printing environment and compensate for things that we know cause bad prints.”
“Some people may say that additive is very mature right now, in general and as an industry, but I don’t think so,” he says, “because Industry 4.0 concepts are really going to take additive to the next level for those technologies that are positioned to take advantage of them.” Q