Since the early 1990s Lithium ion batteries have entered industrial markets as energy storage technology for mobile consumer electronics and battery-operated tools. More recently, Li ion batteries have broken into new markets like battery energy storage systems (BESS) where they are used for load leveling, peak shaving and power demand management in grid-connected and autonomous power networks. Most promising seems to be their use in the market of electric vehicles ranging from electrical bicycles to high-performance passenger cars.
For applications in e-mobility, individual batteries are assembled in a module. Several of these modules are then combined in a so-called battery pack, which among others also comprises systems for cooling and battery management. All these components are integrated in a housing which protects the components inside. In the entire assembly, water ingress may lead to short circuits. In individual batteries, water ingress may lead to capacity loss and generation of hazardous substances due to chemical reactions with battery materials. So leak tightness is a major criterion for stable performance of a battery pack over the expected lifetime of an electrical vehicle.
The requirements for leak tightness often are expressed in ingress protection classes. IPX7 defines protection against immersion of water. This simulates a breakdown scenario in a flooded underpass. IPX9K defines protection against a close-range high pressure water jet representing a cleaning scenario.
IP or Ingress Protection is a term widely used in electronics. It is expressed as a two digit number. The first number refers to its protection against solid objects and runs from 0-6. The second number refers to its resistance to water and runs 0-9. The higher the number, the greater the protection. (see table above)
Many electronics such as smart phones, cameras, headphones, watches, etc., are rated with these IP numbers. The ratings require manufacturers to test their product to ensure that they will pass the test and resist water at the specified depth.
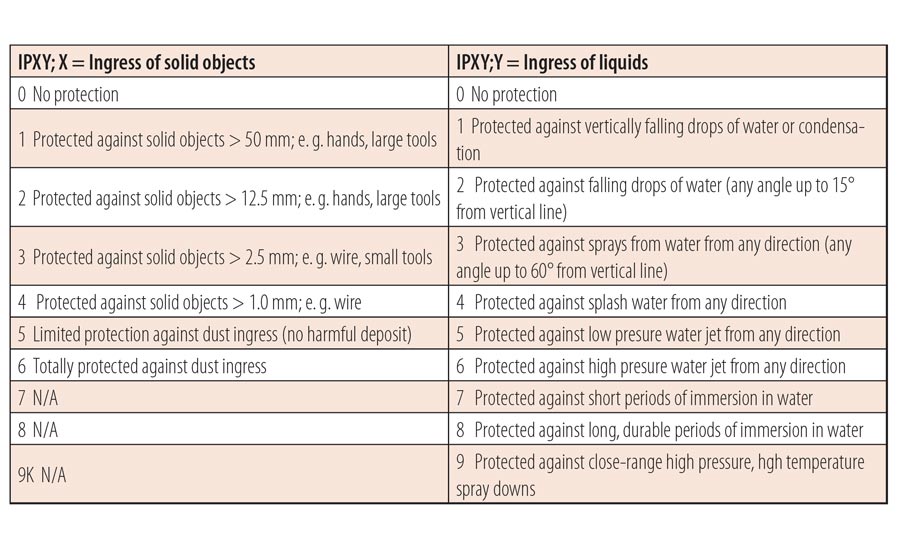
Table 1: Protection against ingress of solid objects and liquids. Click here to see a larger version of Table 1.
Example of common applications:
- Automotive: engine and transmission control modules for major automotive companies and their Tier 1 suppliers
- Fuel/Delivery/Pump Stations: for the electrical enclosures
- Computers components and systems related enclosures
Many manufacturers have conducted their own testing for determining their individual tightness requirements against water ingress or IP protection. Once the rating is verified, one can test the product experimentally by lowering it into a water tank to the specified depth and verifying its functionality. This test is very messy and time consuming. It may be suitable in the R&D phase of a project but not in serial production. The transition from product development to industrial serial production also has to make the transition from a subjective and many times destructive test to a nondestructive, deterministic, and fast test with high gage R&R.
Test method and PASS/FAIL criteria
The phenomenological description of tightness against water ingress has to be translated into an industrial test recipe with selection of test method and PASS/FAIL criteria. From a theoretical point of view a good starting point would be to use the calculation as described in the following paragraph.
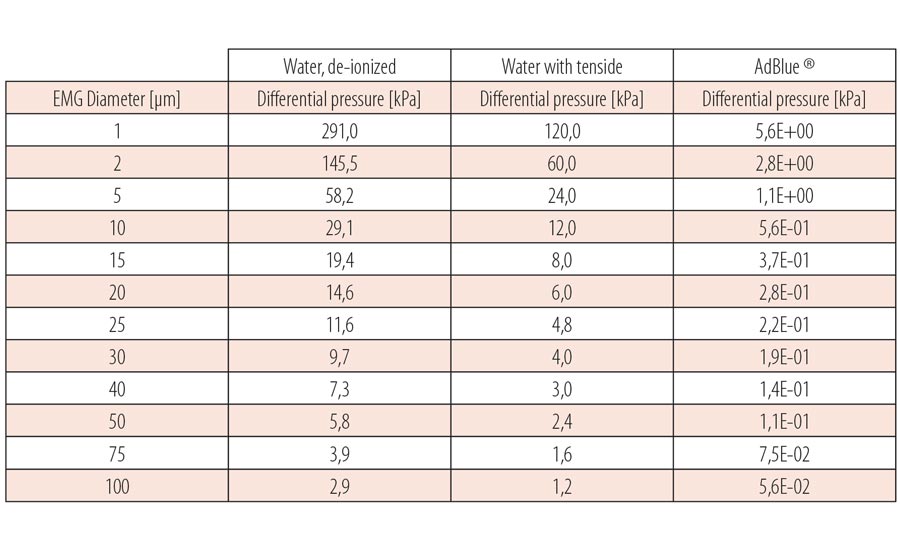
Table 2: Theoretical liquid ingress as a function of defect size and differential pressure (Click here to see a larger version of the table)
How to calculate an ingress protection limit
An IP (INGRESS PROTECTION) limit can be derived using theoretical work by Laplace and Young from the beginning of the 19th century:
Step 1:
Determine pressure or operating pressure from the water depth (2 m Water Column=19.6 KPa; 196 mbar).
Step 2:
Find the smallest diameter that water will not go through with the respective surface tension of the liquid.
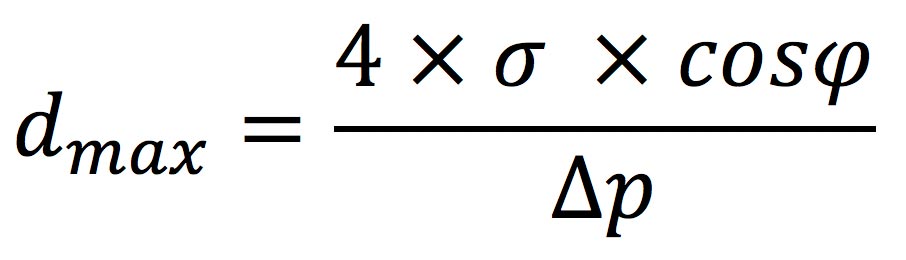
with
dmax Maximum defect diameter [m]
σ Surface tension of the liquid [N/m]
Φ Contact angle of the liquid on the respective surface
Δp Differential pressure [Pa] = [N/m2]
The surface tension of de-ionized water on an Aluminum surface at room temperature is 72.75 E-3 N/m. In many cases the contact angle between the surface of the liquid and the equivalent micro geometry (EMG) cannot be measured with equipment on hand. Hence the cosine of the angle Φ is assumed to be unity (= 1). Table 2 summarizes the resulting pressure gradient needed as a driving force for media transport through a defect as a function of the defect size for various liquid media.
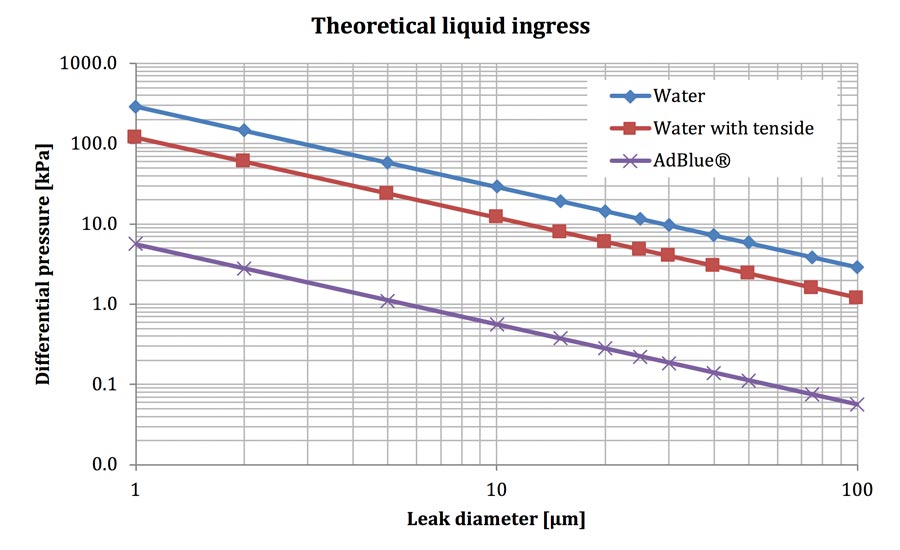
Figure 1: Theoretical liquid ingress as a function of defect size and differential pressure
Step 3:
Define whether the calculated diameter rather fits to an orifice-shaped geometry of the assumed defect (less conservative) or whether a tube-shaped geometry (ED, equivalent diameter for a short wall thickness) better fits to the expected failure model. The latter model leads to a more conservative specification.
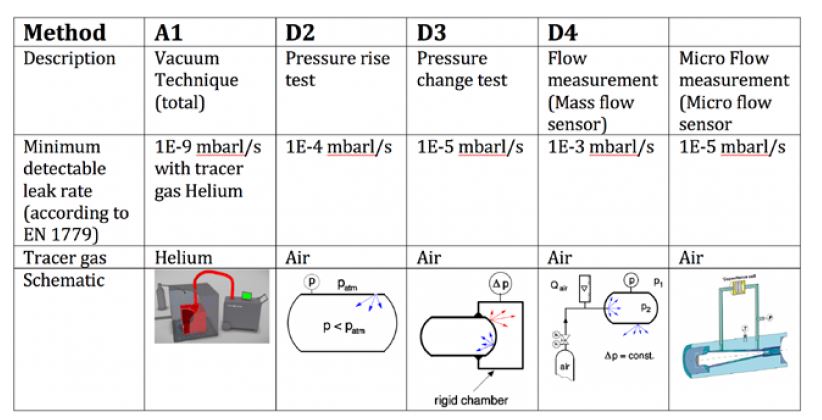
Table 3: Method overview
Data shown in Table 2 and Figure 1 clearly show that surface tension has a massive effect on the pressure needed as driving force to generate liquid transfer through a defect. Surface tension does not only depend on the liquid medium, but also on temperature and wall material. Whereas temperature plays a minor role there is a massive difference whether water flows on the metallic housing, a plastic cooling line or the elastomer seal of a battery pack. For that reason experimental proof for the theoretically derived test recipe is strongly suggested.
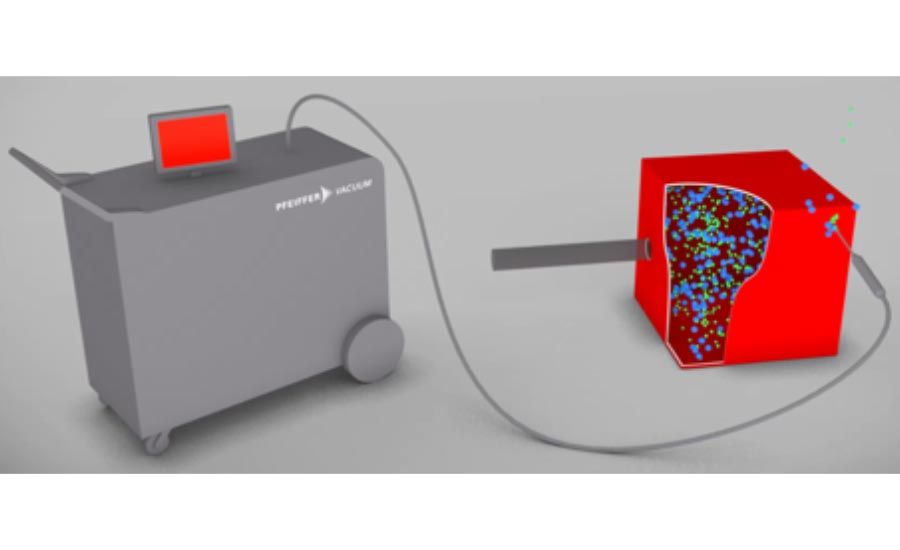
Figure 2: Sniffing method
In addition to the determination of a specification an appropriate test method can be found by applying a procedure suggested in regulations like EN 1779. The steps for selecting the right method are as follows:
Step 1:
Choose the appropriate flow direction for the test
- gas flow out of the object or gas flow into the object
Step 2:
Define the extent of the investigation
- total or local area
Step 3:
Define the aim of the test
- Integral, quantitative leak measurement or qualitative leak location for appliance of a corrective action
Step 4:
Choose the appropriate test method
Step 5:
Check any practical difficulties associated with the test
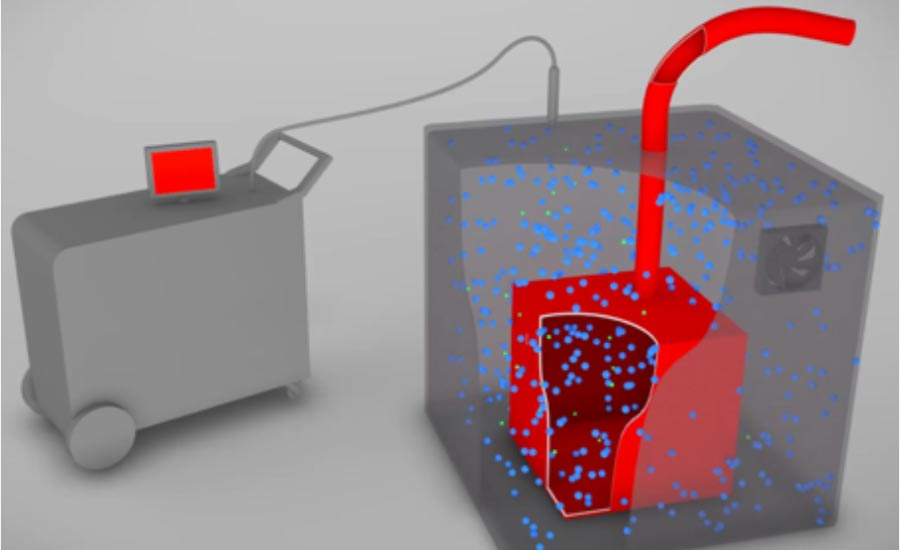
Figure 3: Accumulation method
In our case we would choose a method which detects outside-in and yields a quantitative integral measurement of the total area of the battery pack. The selection matrix in the above-mentioned regulation yields the following methods:
Looking at Table 3 method A1 seems to be the best choice regarding the sensitivity of the method. Since a helium leak detector used for this method is operated in vacuum there are practical challenges associated with this test. Some components inside the battery pack like an encapsulated capacitor do not withstand a high differential pressure. The allowed pressure gradient in real life applications can be as low as 30 mbar. So this method needs to be ruled out since the necessary vacuum for detection cannot be applied.
A pressure rise test can reach the detection limit in the table only with very small volumes and under optimum environmental conditions. Any temperature change (e. g. due to temperature intake from an operator’s hand, a washing step…) or any volume change (e. g. due to flexibility of the battery pack’s top cover) during the test will create a disturbance of the measurement which is larger than the requested detection limit. The pressure change test is the attempt to improve the detection limit of a pressure rise test due to measurement in a smaller volume. However, due to the complicated geometry of a battery pack with fins, connectors, etc., an optimized chamber around the unit under test is not achievable to the needed extent.
Flow measurement seems to have the worst detection limit according to the table above. However, development of micro flow detectors after release of the regulation has pushed the limits of flow measurement to lower detection limits. Sensitivity against environmental influences is not zero with flow measurements but still superior to pressure change measurements. The best results have been achieved by operating the flow detector at a shallow vacuum. Thus ambient pressure provides for a pressure gradient automatically and without any tracer gas cost. The availability of test ports for adaptation of the battery pack often is limited to one or several ports of pressure equalizing membranes. In the light of these practical challenges a micro flow test can be considered as optimum outside-in test method for a battery pack. The free internal volume of the battery pack still acts as a capacitance leading to exponential development of the leak signal over time. A feasibility study has to be performed whether the requested detection limit can be achieved in the given cycle time.
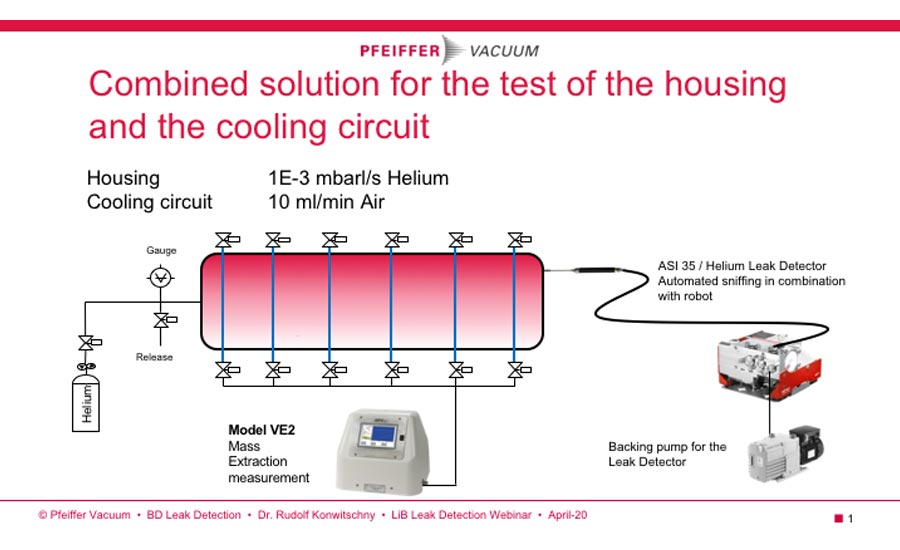
Figure 4: Combined test of cooling circuit with micro flow measurement and battery pack housing with Helium sniffing
Battery pack production still is an emerging technology with failure rates higher than a more mature industrial product. Since the product is at the end of the value-added chain a method for leak location is of interest for purposeful repair.
Leak location using a tracer gas in an outside-in method is impracticable for battery packs since a sniffer probe cannot be brought to the interior sealing area of a battery pack. The practical consequence is testing in reverse direction. This may be a violation of one of the golden rules of leak testing to always simulate the real life pressure gradient of a part during test, but in this case this compromise has to be taken.
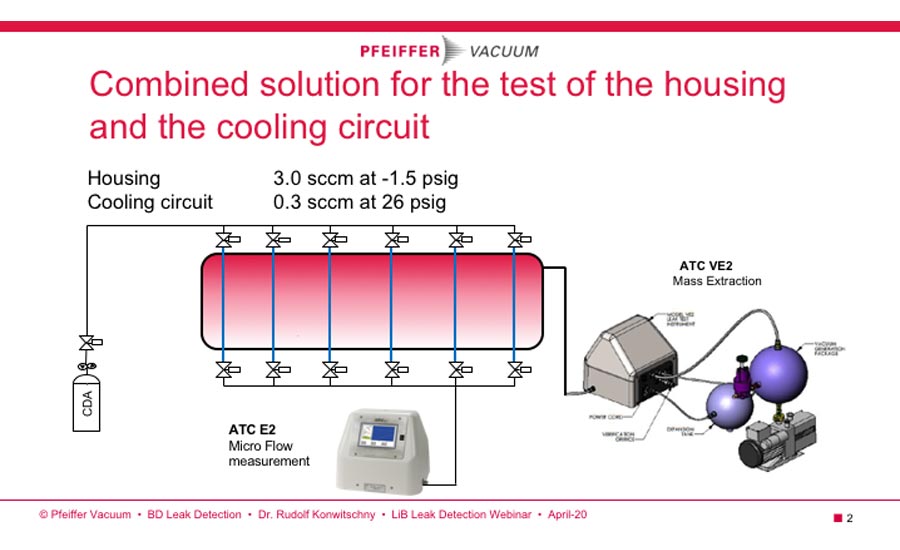
Figure 5: Combined test of cooling circuit and battery pack housing with various micro flow set-ups
For an inside-out sniffing test, the internal volume of the battery pack is charged with tracer gas. If the method is used for qualitative leak location only, there are no special requirements for tracer gas charging or distribution inside the pack. In most cases enough time is available for sufficient gas distribution inside the part. For troubleshooting, manual sniffing is applied with little time pressure on the operator to locate the leak for effective repair.
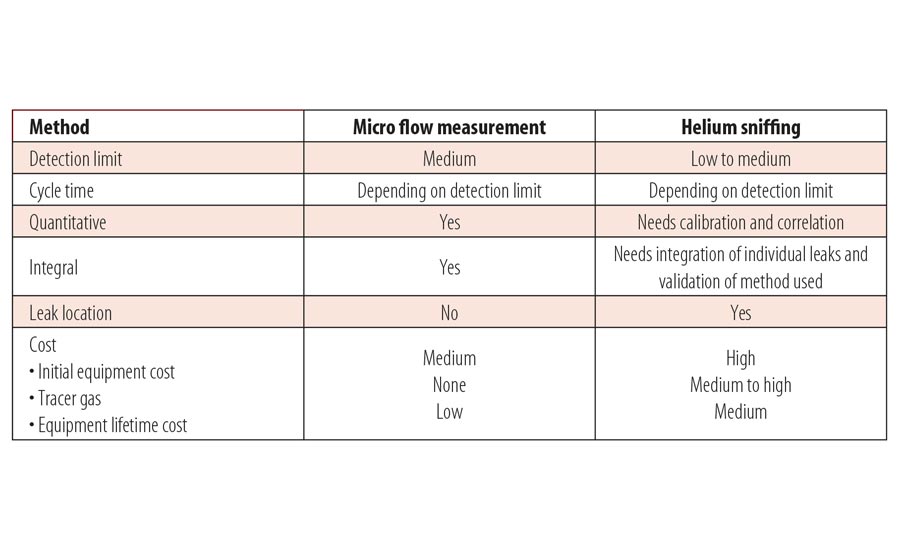
Click here to view a larger version of the table.
In many cases helium sniffing is the only method used in leak testing of a battery pack. This means that special care needs to be taken to adapt this method—which is a qualitative location by definition—to a quantitative measurement. A meaningful quantitative information can only be achieved if the driving force for the tracer gas through a leak is the same or at least conservative at any location of the test area. This means a quantifiable and homogeneous tracer gas concentration inside the part. The concentration achieved inside the battery pack depends on the procedure for tracer gas charging and the geometry of the pack. It is good practice to pre-evacuate the battery pack to its maximum allowed differential pressure and fill it back to the maximum allowed positive pressure. This procedure should be repeated several times in order to stepwise increase the tracer gas concentration inside the pack. The achieved concentration is lower for very rigid packs whereas packs with flexible wall materials (especially the cover) allow for faster build-up of a higher tracer gas concentration. Although it would be desirable to run many cycles, the amount of cycles is determined by the allowed total cycle time for tracer gas charging and test. Special care has to be taken regarding the homogeneity of tracer gas distribution inside the pack. Due to the heavily rugged surface inside the pack any edge will have an influence on the gas distribution. Modularly loaded packs will show different behavior with respect to tracer gas distribution as well as behavior in an integral flow test.
Regarding tracer gas detection, special care has to be taken regarding the velocity of a sniffer probe traveling over the sealing areas of the battery pack and the distance of the sniffer probe. ISO 20485 requests a maximum distance of 1 mm between surface and probe and a traveling speed less or equal 20 mm/s. This is not in line with production requirements. High flow sniffer probes are able to suck in gas from larger distances and with faster traveling times. Still it is strongly suggested to experimentally determine a correlation between tracer gas concentration inside the part, homogeneity of tracer gas concentration, sniffing distance and sniffing velocity. This can be achieved by integration of a calibrated leak at the most unfavorable position of the battery pack seal with respect to the tracer gas charging location. The prepared dummy then can act as a master part in production.
Sniffing can be transferred to a quantitative method by accumulating the escaping tracer gas in a chamber. After homogenization of the gas in the accumulation chamber, the gas can also be quantitatively analyzed with a sniffer probe. However, the speed of this method strongly depends on the volume of the accumulation chamber. Since this volume cannot be minimized to meet all cycle time requirements this method plays a role in low-volume production only.
Finally, the tracer gas which was admitted to the unit under test will not stay inside for an infinite period of time. It needs to be considered whether the test area must be equipped with a purge facility for fast recovery after a massive tracer gas contamination of the workspace.
Practical examples
A quantitative integral leak check of a battery pack can be performed by a micro flow measurement at a pressure slightly below ambient pressure. Air around the battery pack is an infinite and cheap test gas reservoir without any further cost for test gas supply, pre- or post-treatment. A typical test pressure would be 100 mbar below ambient pressure. A detection limit of few sccm can be achieved. Often a combination of cooling circuit leak test is combined with integrity testing of the pack seal. This eliminates the danger that the pre-tested cooling circuits were damaged during production and all joints are tested.
Cooling circuits normally are tested with air. A combination with battery pack testing also is possible with sniffing. In this case a sniffer probe is fed reproducibly around the pack with a robot at constant speed and controlled distance.
Comparison of leak detection methods for battery packs
Although the methods and detectors described in this article have reached a high level of market acceptance and maturity, their appliance to the emerging field of Lithium ion batteries and packs still is on a learning curve. We strongly suggest partnering with an experienced manufacturer of test equipment or plant manufacturer to launch test procedures into the field in the shortest amount of time. NDT