Until the CoaXPress v2.1 standard was released in 2021, the only physical medium between cameras, PCs, and frame grabbers for the CoaXPress (CXP) interface had been 75Ω coaxial cable. For most vision applications, then and now, the CXP 2.1 interface — with its ability to transfer data at up to 12.5 Gbps (Gigabits per Second) per link over a single coaxial cable — perfectly suited high-throughput inspection systems, quality control and factory automation needs. CXP 2.1 over coaxial cable is faster and more stable than GigE Vision, USB3 or Camera Link, and has proven to be the ideal balance between costs and growing requirements for faster speeds, extended length cables, heat dissipation, and power delivery.
So, who would want to change that winning formula?
It turns out the Japan Industrial Imaging Association (JIAA) did, along with the European Machine Vision Association, the Association for Advancing Automation, and a consortium of machine vision companies who make up the CoaXPress standard committee. The committee recognized that bandwidth transmission is rapidly evolving towards 100, 200, and even 400 Gbps, that is, speeds well beyond the capabilities of mere coaxial cables. It amended CXP v2.1 with a light addition that enabled fiber optic links to work with the CoaXPress protocol. With that bold stroke, they introduced the machine vision industry to CoaXPress Over Fiber or CXPoF.
For those unfamiliar with CoaXPress, it was first introduced back in 2007 as an asymmetric, point-to-point serial communication that targeted vision applications requiring high throughput, high-speed transmission of data. The original version (CXP-6) allowed a standard coaxial cable using push-pull DIN connectors to transfer data at rates up to 6.25 Gbps, with an effective data rate of 5.0 Gbps because of 8b10b encoding overhead. When aggregated over two or four links, CXP-6 supported bandwidth up to 25 Gbps from camera to frame grabber. CXP does require a frame grabber unlike GigE Vision applications that use standard Network Interface Cards (NIC). Because GigE Vision relies on a NIC card, data packet management is performed by a driver, while significantly loading the host CPU.
Considering that the Camera Link and GigE interfaces were the ceiling for system speed at the time, CXP was a very welcome innovation. The industry celebrated a host of other attributes, as well, such as more precise triggering, longer cable lengths, and single cable integration for data, control, power (PoCXP), and communications. PoCXP simplified imaging system complexity by eliminating the need to run additional power cables around the project footprint.
CoaXPress is a low latency, low noise interface, which is critical in industrial and medical applications. Industries as diverse as semiconductor and OLED manufacturers, to intelligent traffic systems and biomedical research, quickly embraced the CXP standard. Legacy imaging systems, such as those found on older military aircraft, already had coaxial cable in place for connecting analog cameras. Engineers found it simple to upgrade analog to CXP by swapping out frame grabbers and installing new CXP cameras.
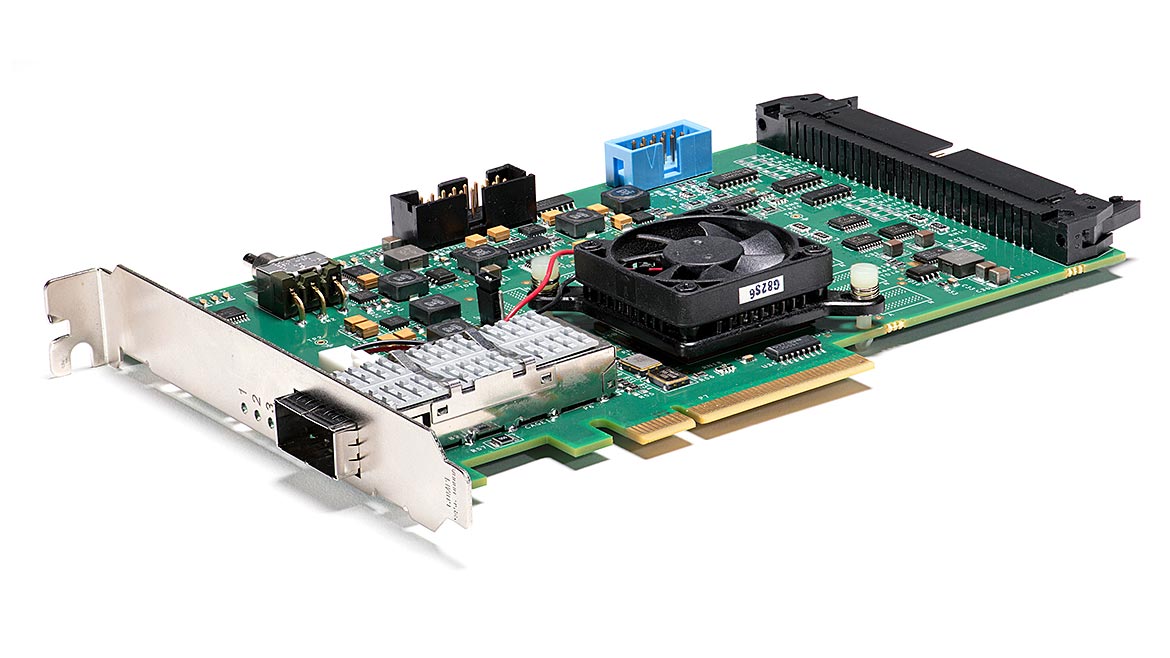
CXP V2.1 Improves The Standard
The CXP v2.1 standard revved up the speed even more. It introduced two blazingly fast new speeds: 10 Gbps (CXP-10) and 12.5 Gbps (CXP-12) that effectively doubled the bandwidth of the original version. Because CXP is scalable from one (single) to two (dual) or four (quad) cables per camera, the CXP 2.1 protocol can reliably achieve bandwidths up to 50 Gbps from one quad link camera if using four cables. CXP-12 comes in ahead of 10GigE and USB 3.1 Gen 2 which can transfer 10 Gbps.
In addition, CXP v2.1 added support for the GenICam generic programming interface and GenDC (Generic Data Container). GenDC is a module that is used to represent, transmit or receive various kinds of data. GenDC targets machine vision related image data, such as 2D, 3D, and multi-spectral, as well as metadata like extra information, histograms and statistics.
Uplink speed has been doubled to 42 Mbps so trigger rates over 500kHz are now possible. The only downside in coaxial cable configurations is that maximum cable length is 35 meters or 115 feet, which limits 50 Gbps transmission to somewhat shorter distances. A new connector type, the Micro-BNC (HD-BNC), replaced the push-pull DIN connector specified in the CXP-6 standard.
Amongst all the excitement about these upgrades, the add-on designed to let the CoaXPress protocol run unmodified over a standard Ethernet connection, including fiber optics, was somewhat overlooked. But not for long. The industry soon realized that CXPoF takes virtually all the advantages of CoaXPress discussed above and combines them with the performance benefits of fiber optic cabling.
What you should know about CXPoF is that it uses Ethernet Layer 1. This layer is where data is physically moved across a network interface. Layer 1 technologies are at the very lowest level of a network. Layer 1 is responsible for the encoding and signaling functions that transform data from bits that reside within a device into signals that can be transmitted over the network. It defines hardware specifications, such as cables, connectors, and network interface cards. Therefore, whenever an upgrade comes to a Layer 1 component, it will directly benefit CXPoF whether that upgrade translates into more bandwidth or lower prices. Compared with machine vision, Ethernet-based computer networking has huge sales volumes, which has driven its component prices down. More economical components, such as cables and connectors, lower the overall price of a CXPoF vision system.
Higher Bandwidth
CXPoF’s net bandwidth matches CXP-12—approximately 40 Gbps. However, instead of requiring four CXP-12 links and four coaxial connections, CXPoF transmits 40 Gbps on a single QSFP+ (Quad Small Form Factor Pluggable) transceiver module and a single fiber optic cable. This translates into a far less complex cabling structure with fewer space requirements. As speeds increase further from 12.5 Gbps to the JIIA’s expected 25 Gbps via QSFP28 and 50 Gbps via QSFP56 per lane, CXPoF appears to be the path of least resistance.
Why Fiber Optics?
Fiber optics transmit data as pulses of light through strands of fiber made of either glass or plastic. When bundled with hundreds of other strands, the resulting fiber optic cable will transmit more data at faster speeds and over much longer distances than high-grade copper cables. Also, fiber optics are electrically isolated. Accordingly, it does not radiate, and it is not susceptible to electromagnetic interference (EMI) that can slow transmission speeds or create crosstalk. Fiber optic cable isolation eliminates problems associated with grounding as well.
Fiber optic cables offer several other advantages. For one, fiber optic cables do not represent a fire hazard as heat is not dissipated. Second, the cables can withstand significant pull forces without suffering breakage over long runs compared to copper cabling. Third, fiber optic cables are not damaged by inclement weather, moisture, or temperature extremes to the same extent as coaxial or Ethernet cables. Finally, being lighter and smaller in diameter than other cable types, fiber optic cables can be easily threaded on machines, robots, aircraft, and ground vehicles.
There are two types of fiber optic cables: Single Mode Fiber (SMF) and Multi-Mode Fiber (MMF) with the difference being the size of the core. MMF has a much wider core, allowing multiple modes or “rays” of light to propagate. SMF has a very narrow core, allowing only a single mode of light to propagate. In general, SMF cable is for extremely long-distance signal transmission, while applications requiring a larger volume of data to be sent over a shorter run tend to use MMF cabling. CXPoF is expected to achieve distances up to 80 km (about 49.71 mi) in single-mode and 300 m (about 984.25 ft) in multi-mode without the use of error-prone extenders.
Perhaps the only downside to CXPoF is the loss of the ability to send power. PoCXP notwithstanding, CXPoF keeps and builds on all the benefits of the CXP protocol.
Moving From Coax To Fiber
As an extension of the CoaXPress protocol, CXPoF provides the generic GenICam programming interface for hardware and software, plus is independent of any operating system, making it simple to upgrade from coaxial to fiber optics. Upgrading will require CXPoF-compliant cameras and frame grabbers, as well as fiber optic cabling, transceivers, and connectors. Vendors are beginning to recognize the market potential of CXPoF. Consequently, hardware choices are limited now but are projected to grow exponentially as has been the case with previous CXP upgrades.
Vision & Sensors
A Quality Special Section
A CXPoF frame grabber will have a fiber transceiver slot supporting QFSP+ compatible fiber cable assemblies. The assembly will provide up to four connections to support one quad link camera, two dual link cameras, or four single link cameras. CXPoF frame grabbers use the same half-Size x8 PCI Express Gen 3.0 platform as CXP frame grabbers, making it simple to swap out in the host PC. The optical transceiver module consists of a receiving end and a transmitting end. The receiving part realizes a photoelectric conversion, and the transmitting part realizes an electrooptical conversion.
A single fiber optic cable can achieve speeds of 40 Gbps. This means that fewer cables are required to transmit data, reducing the complexity of the cable run, lowering system costs, and minimizing potential failure points, especially if fiber extenders were previously required.
Conclusion
CXPoF opens a new realm of opportunities. It is likely that the first applications to take advantage of CXPoF will be in situations requiring exceptionally long cabling such as ITS or ballistics testing, or when electrical isolation is required between the camera and the PC as is the case in noisy industrial sites and sensitive medical environments.
In addition, as mentioned, economics will play a role in the pace at which CXPoF is adopted. CXPoF has cost benefits over traditional coax because of its ability to use Ethernet networking components, especially as this applies to cable costs. For example, a 30M quad CXP-12 coaxial cable costs over $600, while a 30M QSFP+ AOC (Active optical cable) costs about $125. Combined with its other advantages, this cost effectiveness might be enough to convince system integrators to migrate to CXPoF.
The roadmap for CXP-over-Fiber is exciting. Besides supporting extremely high throughput today it is uniquely poised for future bandwidth increases, all while using common, low-cost components.